Cosmic Inflation
Cosmic inflation is an exponential expansion of space that is thought to have occurred between 10-36 and 10-34 seconds after the opening of the universe. It is thought to have preceded the Big Bang, the explosive expansion of the early universe, and was a more rapid expansion phenomenon.
Inflation is a powerful theory for solving problems in modern cosmology, such as the uniformity and flatness of the universe, which cannot be explained by the Big Bang theory. However, its existence has not yet been confirmed by observations.
According to the theory, gravitational waves are the result of an expansion so rapid that it has been described as “an amoeba growing to the size of a galaxy in an instant”. These are called Primordial Gravitational Waves (PGWs).
If we can directly observe these PGWs, we can say that we have proved the inflation theory correct. However, PGWs are very weak and therefore difficult to observe directly.
Therefore, cosmologists try to detect PGWs “indirectly”. According to inflationary theory, the electromagnetic waves from the full-sky, called the Cosmic Microwave Background (CMB), have a special polarization pattern, called B-mode polarization, which is imprinted by PGWs.
The goal of the Ishino laboratory is to find the B-mode polarization and verify the inflation theory, thereby revealing the beginning of the universe.
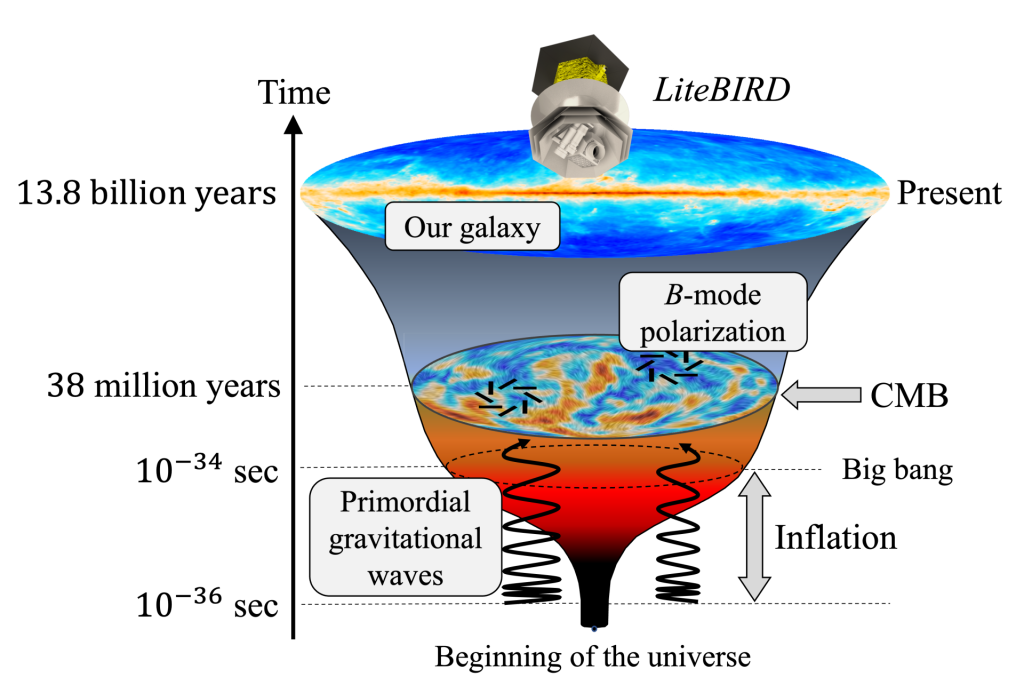
Cosmic Microwave Background
The Cosmic Microwave Background (CMB) is electromagnetic radiation in the microwave and millimetre-wave bands, emitted from all directions in the universe. It is characterized by the fact that it can be observed with uniform intensity throughout the sky, and that its spectrum matches very well that of blackbody radiation. These features provide strong evidence for the Big Bang theory, which states that the Universe was once a hot, dense fireball that gradually cooled to its present shape as space expanded.
The CMB, also known as “the oldest light in the universe” because it is the electromagnetic wave from the time when electromagnetic waves could first travel in a straight line, is an extremely important observational target in modern cosmology because it contains a great deal of information. In recent years, high-precision observations of the CMB by the Planck space telescope have revealed that the universe we live in is 13.8 billion years old.
As mentioned above, the CMB is also thought to be imprinted with the effects of primordial gravitational waves caused by cosmic inflation in the form of B-mode polarization. However, B-mode polarization is weaker than the CMB’s temperature fluctuations and other polarization components, known as E-modes, and therefore requires 100 times greater sensitivity than conventional polarization to observe. CMB observing projects are currently underway around the world to develop and exploit more precise experimental instruments and analysis methods.
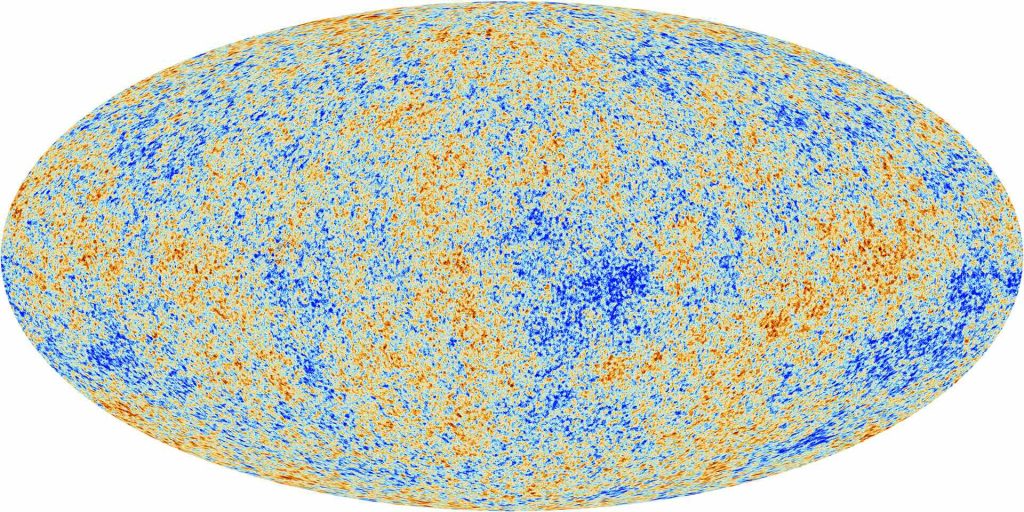
LiteBIRD
LiteBIRD is a JAXA-led space telescope project that will observe the CMB over the entire sky in the microwave and millimetre-wave bands from 34-448 GHz for three years. LiteBIRD will be the world’s first and most sensitive space telescope for B-mode observations of the CMB and is scheduled for launch in 2032. The intensity (tensor scalar ratio r) of the primordial gravitational wave, which is the origin of the B-mode, can be estimated from LiteBIRD observations, making it possible to identify the inflationary model that shaped our universe.
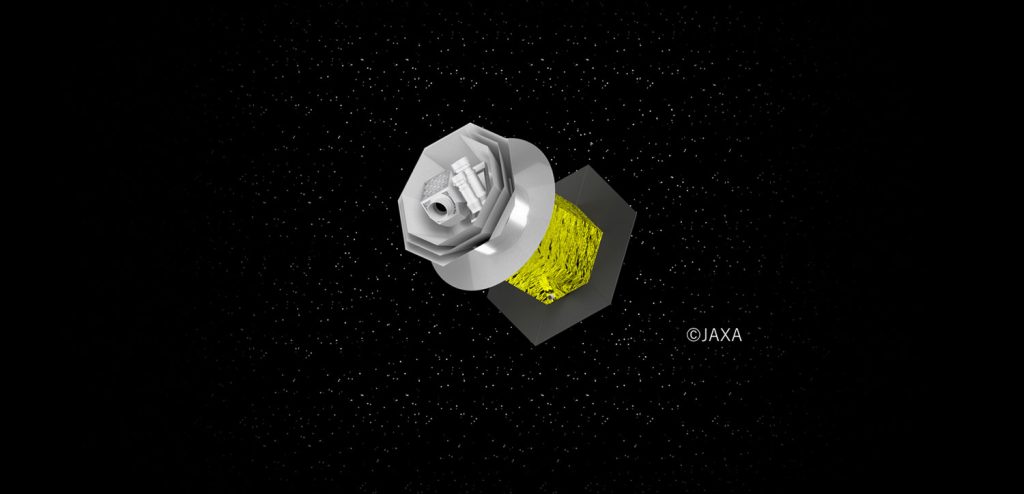
Systematic errors
Within the LiteBIRD collaboration, which is a joint effort of universities and research institutes around the world, we at the Ishino Laboratory are responsible for “systematic error analysis”. Systematic errors are errors that originate in the measurement instruments, such as the discrepancies in the scale itself. Unlike statistical errors, which become smaller as the number of measurements and the measurement time increase, systematic errors do not essentially disappear even if measurements are made carefully over a long period of time. However, if the discrepancies in the scale can be detected in some way and the magnitude of the discrepancy can be known, it may be possible to reduce or eliminate the effect of the discrepancy.
In LiteBIRD, various systematic errors and their contributors, such as detector gain and uncertainty in the direction of observation, are taken into account. We have developed a mathematical model for the systematic errors. We are also developing new data analysis methods that use advanced mathematical techniques to separate the true signal from the systematic errors and improve the accuracy of CMB polarization observations.
GPU
GPUs (Graphics Processing Units) are also used for numerical calculations related to systematic errors. CMB data analysis requires an enormous number of calculations to be performed quickly and efficiently. Because of its high parallel processing capacity, the GPU can perform many calculations simultaneously, dramatically increasing the efficiency of large, complex calculations and allowing calculations that would take a conventional CPU a long time to complete to be performed quickly. This significantly reduces analysis time and enables more efficient CMB data analysis.
Foregrounds
Microwaves from many different sources exist in the Universe, not just the CMB. These are called “Foreground” because they exist in the foreground, while the CMB is in the background; when observing the CMB, the foregrounds are mixed in, so it is necessary to separate or remove this foregrounds. Because the intensity of the foreground is very strong relative to the CMB, a method to separate or remove the foreground with high accuracy is required to observe the CMB. There is a wide range of separation and removal methods, but they basically take advantage of the fact that the CMB and the foreground have different properties. To validate cosmic inflation, the polarization of the CMB must be measured with high precision. For this reason, our research aims at high-precision separation and removal of the CMB and the foregrounds.
Solar neutrinos
The sun emits light as a result of the fusion of hydrogen into helium at its center. This fusion process begins when two protons collide, producing deuteron, positron, and electron neutrinos (pp reaction). The neutrinos produced in this reaction are known as pp neutrinos: measuring pp neutrinos can provide valuable information about the state of the Sun’s interior that cannot be observed with electromagnetic waves. In 1976, Raghavan proposed the use of 115In (indium) as a target [PRL 37, 259, 1976]. This allows the excitation of gamma rays by delayed coincidence to significantly reduce background events. We develop a novel detection system combining an indium containing target with a superconducting detector to observe solar neutrinos using unconventional methods.
Superconducting Detector MKID
MKID (Microwave Kinetic Inductance Detector), a type of superconducting detector, is used to observe neutrinos. Inside a superconductor, two pairs of electrons called Cooper pairs are formed. These Cooper pairs are the origin of superconductivity, which can be destroyed even at very low energies due to their weak binding energy. MKID is a superconducting detector that forms a tiny LC resonator with a superconducting thin film and reads microwave signals.
When external energy enters the resonator and breaks the Cooper pair, the resonant frequency changes, and the energy is detected by reading the change. Since neutrinos are elementary particles that do not easily interact with each other, a large target is required for detection. Detectors must be placed throughout this large target and constantly observe them.
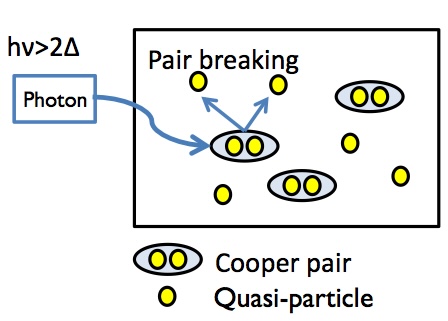
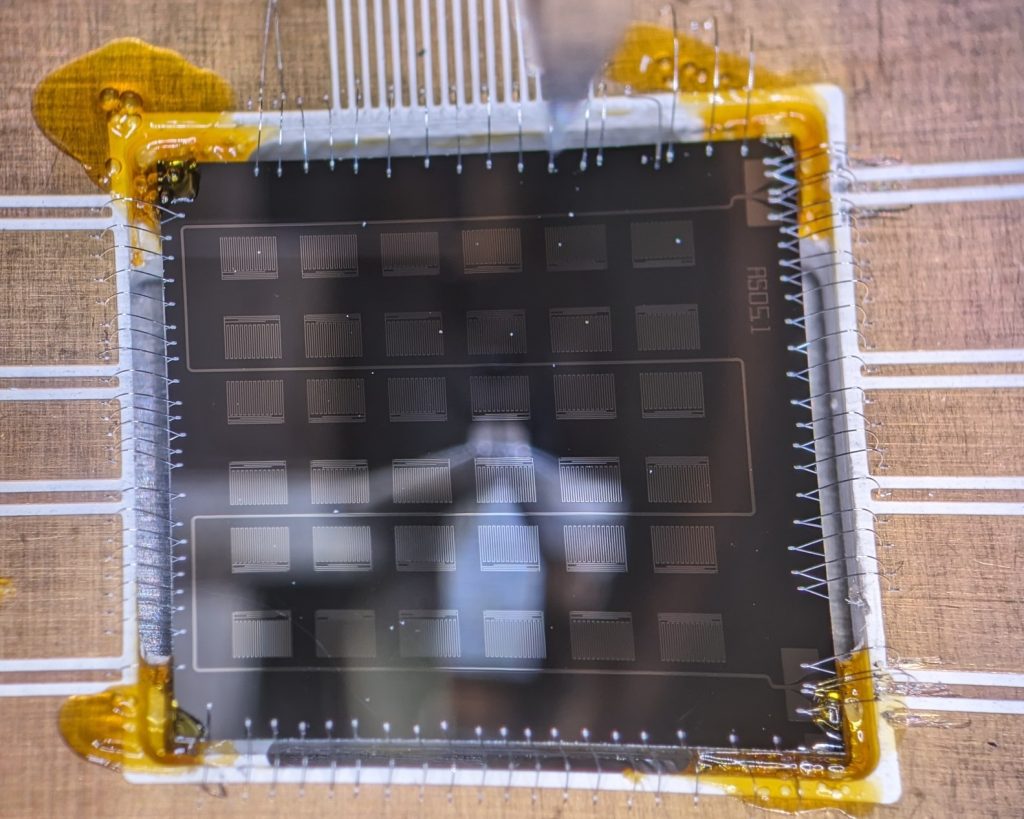